The work of the Structural Biology Laboratory is internationally recognised. Our work has defined more than 200 crystal structures and more recently, structures derived from cryo-election microscopy – including those of membrane-associating proteins, detoxifying enzymes and protein kinases. This work has provided insights into cancer, bacterial and viral infections, and neurological diseases such as Alzheimer’s disease.
In recent years, we have emphasised the translational aspects of our work, with increasing focus on structure-based drug discovery. This focus has been underpinned by development of virtual screening and fragment screening platforms in-house, by funding from the Australian Cancer Research Foundation, and partnerships with biotechnology companies including CSL Limited and Janssen.
Current research projects
-
Structural biology of neurodegenerative diseases
Microglial receptors
Microglial cells, the resident immune cells of the central nervous system, act as the first and main form of active immune defence in the brain. Upon detection of pathogens or damage, microglia adopt an activated state resulting in an inflammatory response which leads to the release of a host of neuroactive signalling molecules. However, in neurodegenerative diseases these cells fail to remove the large excess of neurotoxic proteins that accumulate in the brain. Receptors expressed on the surface of microglia play a role in sensing environmental changes and regulating their activation. We are exploring the role of various microglial receptors in normal physiology and in neurodegenerative diseases, with the aim of developing new drug treatments.
MER tyrosine kinase (with Prof Jonathan Baell, Monash Institute of Pharmaceutical Sciences)
MER tyrosine kinase (MERTK) upregulation is associated with activation of microglia and plays a vital role in neuroregeneration following damage induced by neuroinflammatory diseases such as multiple sclerosis (MS). A radiotracer specific for MERTK could be of great utility in the clinical management of MS, for the detection and differentiation of neurodegenerative and neurodegenerative processes. This project aims to develop theranostics – diagnostics and drugs – to treat neurodegenerative diseases.
Amyloid Precursor Protein
Alzheimer’s disease is the most prevalent neurodegenerative disease in humans and is the fourth leading cause of death in the developed world. The disease is characterised by the presence of amyloid plaques that principally derive from amyloid precursor protein (APP). The long-term aim of this project is to determine the complete structure of APP, in order to understand its normal physiological function and as a basis for structure-based drug design of anti-Alzheimer’s drugs.
Antibodies and diabodies (with Prof Peter Crack, The University of Melbourne)
A widely advocated clinical strategy to treat Alzheimer’s disease is to use antibodies to remove neurotoxins, Abeta and hyper-phosphorylated Tau (pTau), that are thought to contribute to the disease. Our lab has visualised, by crystallography, how three such antibodies recognise the Abeta peptide, including the clinical antibodies Bapineuzumab and Solaneuzmab. In a different approach, we have developed bispecific diabodies which consist of one arm with specificity for a pathogen (Abeta or pTau in Alzheimer’s and α-synuclein in Parkinson’s) and the other arm which recognises a microglial surface protein that drags the pathogen into microglia for degradation.
Structural biology of cancerEpigenetic regulators (with Prof Jonathan Baell, Monash Institute of Pharmaceutical Sciences)
Breast cancer is the most frequently diagnosed cancer globally, with oestrogen receptor positive (ER+) breast cancer being the most common subtype, representing 84% of cases. Increasing resistance is observed with existing drugs; hence, there is a need to develop new drugs with novel mechanisms of action. Our project focuses on finding drugs for ER+ breast cancer by targeting KAT6A, a key enzyme responsible for the development of breast cancer. Our objective is to provide a proof-of-concept of the validity and feasibility of KAT6A inhibitors (KAT6Ai) and degraders (KAT6Ad) as paradigm-shifting treatments of ER+ breast cancer.
People
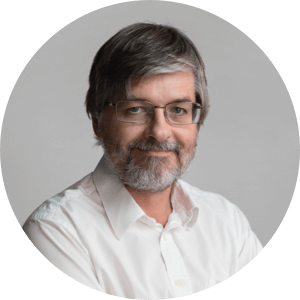
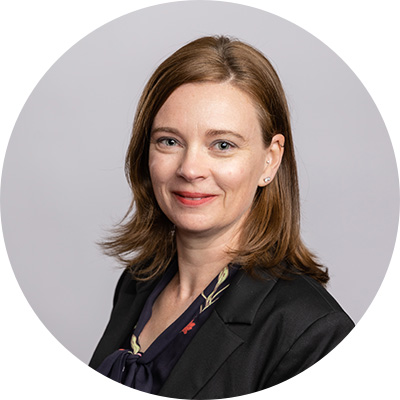
Lab Manager and Senior Research Officer, Structural Biology Lab
View Profile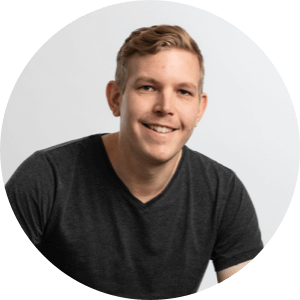
- Dr Tracy Nero, Research Fellow
- Gabriela Crespi, Research Assistant
- Nancy Hancock, Research Assistant
- Dr Julian Adams, Research and Development Manager, Brookhaven National Laboratory, USA
- Dr Chris Anstey-Gilbert, CEO, BioEmergence, UK
- Professor David Ascher, Deputy Director of Biotechnology, University of Queensland
- Dr Brett Bennetts, Project Manager, The Florey Institute of Neurosciences and Mental Health
- Dr Sophie Broughton, Senior Program Advisor, Victorian Cancer Agency
- Dr Brett Cromer, Senior Lecturer, Swinburne University of Technology
- Dr Urmi Dhagat, Manager, The University of Melbourne
- Associate Professor Michelle Dunstone, Lab Head, Monash University
- Dr Guido Hansen, Group Leader, University of Lübeck, Germany
- Dr Jessica Holien, Research Fellow, RMIT
- Dr Jack King-Scott, Director, Kings Patent & Trade Mark Attorneys
- Dr Belinda Michell, Protein Production Specialist, The University of Melbourne
- Dr Craig Morton, Principal Research Scientist, CSIRO
- Associate Professor Aaron Oakley, Research Fellow, University of Wollongong
- Dr Lorien Parker, Owner, SciencePlay Kids
- Professor Jamie Rossjohn, Head of Infection and Immunity Program, Monash University
- Dr Peter Walsh, Director, Yarra Trail Medical
- Dr Jerome Wielens, Director, Health and Life Sciences, Breakthrough Victoria
- Professor Matthew Wilce, Lab Head, Monash University
Student projects
Structural biology of proteins involved in neurodegenerative disorders and mental illness
Lab: Structural Biology
Supervisor(s): Professor Michael Parker
Diseases focus: Healthy AgeingSelected publications
Parker, M.W., Buckley, J.T., Postma, J.P., Tucker, A.D., Leonard, K., Pattus, F. & Tsernoglou, D. (1994) Structure of the Aeromonas toxin proaerolysin in its water-soluble and membrane-channel states. Nature 367, 292-295. DOI: 10.1038/367292a0
Rossjohn, J., Feil, S.C., McKinstry, W.J., Tweten, R.K. & Parker, M.W. (1997) Structure of a cholesterol-binding, thiol-activated cytolysin and a model of its membrane form. Cell 89, 685-692. DOI: 10.1016/s0092-8674(00)80251-2
Rossjohn, J., Cappai, R., Feil, S.C., Henry, A., McKinstry, W.J., Galatis, D., Hesse, L., Multhaup, G., Beyreuther, K., Masters, C.L. & Parker, M.W. (1999) Crystal structure of the N-terminal, growth factor-like domain of Alzheimer’s amyloid precursor protein. Nature Struct. Biol. 6, 327-331. DOI: 10.1038/7562
Hansen, G., Hercus, T.R., McClure, B.J., Stomski, F.C., Dottore, M., Powell, J., Ramshaw, H., Woodcock, J.M., Xu, Y., Guthridge, M., McKinstry, W.J., Lopez, A.F. & Parker, M.W. (2008) The structure of the GM-CSF receptor complex reveals a distinct mode of cytokine receptor activation. Cell 134, 496-507. DOI: 10.1016/j.cell.2008.05.053
Miles, L.A., Crespi, G.A.N., Doughty. L. & Parker, M.W. (2013) Bapineuzumab captures the N-terminus of the Alzheimer’s disease amyloid-beta peptide in a helical conformation. Sci. Rep. 3, 1302. DOI:10.1038/srep01302
Miles, L.A., Hermans, S., Crespi, G.A.N., Gooi, J.H., Doughty, L., Nero, T.L., Markulić, J., Ebneth, A., Wroblowski, B., Oehlrich, D., Trabanco, A.A., Rives, M-L., Royaux, I., Hancock, N.C., & Parker, M.W. (2019) Small molecule binding to Alzheimer’s risk factor CD33 promotes Abeta phagocytosis. iScience 19, 110-118. DOI: 10.1016/j.isci.2019.07.023
ORCID profile: https://orcid.org/my-orcid?orcid=0000-0002-3101-1138
Google Scholar profile: https://scholar.google.com/citations?user=ZrUEdmcAAAAJ&hl=en